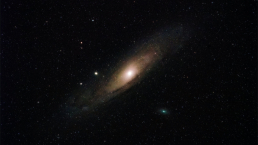
Mapping the Universe with Nobel Prize Winner George Smoot
Prof Dr George Smoot played a key role in the breakthrough that solidified the Big Bang theory’s place in cosmology. For this, he won the Nobel Prize in Physics 2006. Here, we’ll go over his fascinating career and explore the work he publishes with MDPI.
Who is George Smoot?
George Smoot is an active researcher in observational astrophysics and cosmology at the University of California, Berkeley, and Laurence Berkeley National Laboratory. His group are observing our galaxy and the cosmic background radiation that can inform us about the beginning of the universe. This includes ground-based radio‒telescope observations, balloon-borne instruments, and satellite experiments.
Cosmology is basically the study of the cosmos; it aims to provide an accurate description of the universe. Smoot entered cosmology when there were still many open questions about it, relating directly to fundamental questions about the structure of the universe.
Winning the Nobel Prize in Physics for COBE
George Smoot won the Nobel Prize jointly with John C. Mather for developing the NASA Cosmic Background Explorer (COBE) satellite.
Various types of particles and radiation travel through outer space, including cosmic background radiation (CMB). COBE measured miniscule variations in CMB which supported the hypothesis that the universe emerged from a state of high temperature and density, i.e., the Big Bang. The variations represent the seeds of the planets, stars, and galaxies that exist now.
Significantly, Stephen Hawking called this the “discovery of the century, if not all time”. Alongside, the Nobel Prize in Physics, Smoot has been awarded the NASA Medal for Exceptional Science Achievement, Kilby Award, and Lawrence Award.
Exploring the research of George Smoot
George Smoot has published various articles and reviews with MDPI’s journal Universe, and he is an Advisory Board Member of the journal.
Firstly, we will go through two of his reviews, which provide historical perspectives into the field of cosmology. These provide deep insights into the field and its development, and more broadly about the nature of scientific discovery itself.
In the second review, he explains how he is interested in writing for scientists outside the fields of astrology and cosmology. By publishing such work as Open Access, Smoot enables researchers from other fields to access the insights of his disciplines and build from them. From this, cooperation and innovation can flourish.
Finally, we will discuss an article that explores some of the open questions remaining in the field.
The history of gravitational waves
Basically, gravitational waves are invisible and rapid ‘ripples’ in space caused by an intense event, like a star exploding or two black holes merging. Smoot reviewed the history of our understanding of gravitational waves from Einstein’s predictions to their eventual discovery.
First, he describes how Albert Einstein’s General Theory of Relativity (GR), published in 1915, predicted them. However, Einstein expected them to be very faint, and doubted that they could ever be observed. This preoccupied Einstein in the decades after publishing this work, wondering whether they were just a formal mathematical construct with no real physical meaning.
In the 1960s, efforts ramped up to detect gravitational waves. Many scientists developed their own detectors across the world: hopes were raised, and often they were discredited or falsified by research.
For example, Joseph Weber and his team spent several years developing a tool which supposedly detected gravitational waves from the centre of the Milky Way. His results implied a rapid decrease in the mass that gravitationally kept our galaxy together. If this were the case, our galaxy would have already been dispersed many years ago.
The team were detecting very sporadic events, including other forms of radiation. Nonetheless, Weber was considered a pioneer and set an example for many of the subsequent tools that were developed.
At the end of the century, however, the Laser Interferometer Gravitational Wave Observatory (LIGO) combined many of the innovations of its predecessors.
Detecting gravitational waves
LIGO had observatories located diagonally opposed across the US, in Hanford and Livingston, 1900 km apart. After upgrades through the 2000s, LIGO began operating again in 2015. Very quickly, a signal arrived. This signal had travelled for over a billion years, coming from a pair of black holes merging and emitting gravitational radiation.
The waveform detected by both LIGO observatories matched Einstein’s predictions almost exactly 100 years later. This represented one of the final unproven predictions of GR.
Smoot’s account of the discovery of gravitational waves highlights the role of theory in science, and how far theory can go before it is eventually proven. Tackling complex issues like understanding our universe requires persistence, collaboration, and ambition. By moving back and forth between theory and practice, the countless scientists involved in this story opened a new window of observation for the universe.
What is the Hubble constant?
One of the main uncertainties in modern cosmology is the present rate of the universe’s expansion, which is known as the Hubble constant. However, different observational techniques bring about different results, causing what is known as Hubble tension. Some suggest that Hubble tension points to something missing from our understanding of the cosmos, which could have implications for our fundamental understanding of physics.
Let’s explore a review co-written by George Smoot that overviews the history of the Hubble constant from the beginning of the 20th century to the present.
Measuring the size of the universe
In 1920, a debate between two scientists on the size of the universe showed the diverging viewpoints. One maintained that the Milky Way was the entire universe, whilst the other suggested that our galaxy was one of many in a larger universe. The root of the debate was the problem of determining distances between celestial objects, namely, how far away Andromeda is. Andromeda is the nearest neighbouring galaxy to the Milky Way.
Then, later in the 1920s, Edwin Hubble demonstrated that Andromeda was far outside the Milky Way by measuring light from a star with varying brightness. Alongside this, Vesto Melvin Slipher discovered that many galaxies were rapidly moving away from Earth, whilst a few others were approaching.
Debates persisted through the 20th century, with Hubble and others determining ways to measure distance and ultimately represent the size of the universe with the Hubble constant.
An open question about the universe
At the end of the 20th century, a revaluation of the most reliable measurements for cosmic distance determination occurred.
Basically, there are two ways to determine the Hubble constant. One involves measuring features in the relative recent universe, and another from using the CMB from shortly after the Big Bang. Each result provides a set of results that are very similar. Thus, we have two differing groups of results that are apparently correct.
This has created an unsatisfactory and uncomfortable situation in cosmology. Smoot concludes by explaining how new experiments, theories, and observations are needed. But will these help to determine the Hubble constant or create more tension?
Undeniably, this narrative goes to show the necessity of grappling with uncertainty in science. Such questions require looking both backwards and forwards. And accepting that the answers may lead to some unwanted truths, ones that may challenge ideas and concepts we have taken for granted.
More unsolved questions about the universe
Finally, Smoot dedicates an article exploring more of these open questions and pointing towards future directions in cosmology. Specifically, he looks at areas where we may need to replace or revise GR.
All gravitational phenomena that have ever been observed can be modelled by GR. This includes falling apples, the orbit of planets, and even black holes. However, there are some things GR cannot account for:
- The accelerated expansion of the universe.
- The presence of a mysterious form of matter that cannot be observed directly.
- The initial conditions of the early universe.
Exploring these questions could reshape our fundamental understanding of physics.
Our expanding universe
Applying GR to the universe helped develop the Hot Big Bang model: the universe is expanding out of an initial highly energetic, dense state. But there are observable regions of the universe that are further than the speed of light could have travelled since the Big Bang.
So, this led to a modification of our understanding: that there was an inflationary phase after the Big Bang.
Nevertheless, another question opened: why is the universe so flat? There is no magnetic monopole that would make it so.
So, the model was further developed to suggest that the universe is undergoing a period of exponential accelerated expansion from the Big Bang, which lends its flatter shape and explains how its size exceeds the speed of light.
Dark matter
In the 1920s, astronomers noticed the amount of visible matter in galaxies didn’t add up to their rotational curves. This implied that the amount of mass in galaxies is much higher than is visible.
Presently, this is known as dark matter. Dark matter accounts for around 25% of the mass content of the universe. However, many models of it are unstable and require actions that depend on the mass of the galaxy being investigated, thus providing a different theory for each galaxy.
Dark matter remains a mysterious and elusive property of the universe, complicating our understanding of matter.
What’s next in cosmology?
So, Smoot suggests, we need to observe the evolution of the universe and check whether it corresponds to the predictions of GR, specifically whether cosmic acceleration and the formation of dark matter line up.
Now is a critical point for this. Computing power and data are catching up to the theory. Artificial intelligence could provide us with insights that far exceed our capabilities.
However, as mentioned, answering these questions will force us to face even more questions about fundamental physics:
- Are there forces and interactions besides the four we know of (gravity, electromagnetism, and the strong/weak nuclear forces)?
- Are there particles beyond the Standard Model?
- What is the real structure of spacetime?
- Are there extra dimensions?
By publishing this work as Open Access, Smoot is opening out these questions to the global scientific community. That way, we can all collaborate and deal with the implications of gaining such profound insights.
Understanding the cosmos with George Smoot
George Smoot is an innovator and leader in studying cosmology. We are delighted that he chooses to submit work to MDPI.
Universe is an international peer-reviewed Open Access journal focused on principles and new discoveries in the universe. It includes 14 sections, including Cosmology, Gravitation, and Field Theory.
The journal has also published work by Prof Gerald t’ Hooft, who won the Nobel Prize in Physics 1999 for his work on electromagnetic interaction. Additionally, Prof Roger Penrose, winner of the Nobel Prize in Physics 2020 for his work on black hole formation, serves as an Advisory Board Member. If you want to learn more about the journal, see our Spotlight on Universe.
If you’re interested in submitting, it will have a section for you. See the journal homepage if you are considering submitting your work.